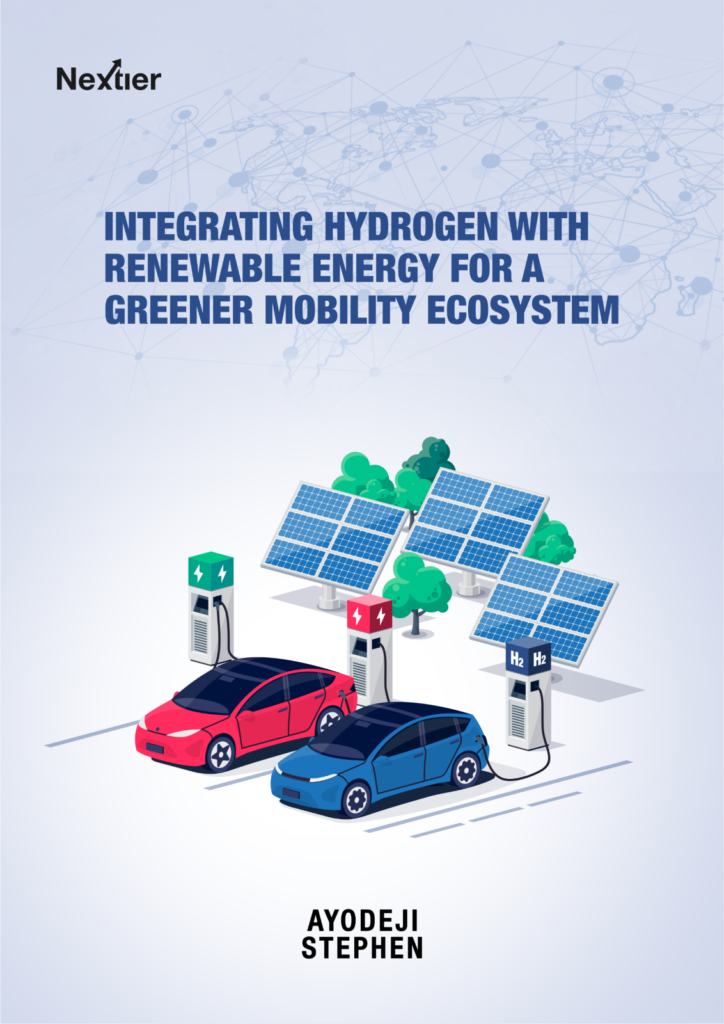
Integrating hydrogen with renewable energy sources is an essential strategy for achieving a greener mobility ecosystem in the quest for sustainable development and combating climate change. Hydrogen holds immense potential as a clean and scalable energy carrier due to its high energy density and zero emissions when used in fuel cells to produce electricity. Unlike fossil fuels, hydrogen combustion produces only water vapour, making it a compelling alternative for reducing greenhouse gas emissions and air pollution associated with conventional transportation. Integrating hydrogen with renewable energy sources, such as wind, solar, and hydroelectric power, is important for ensuring its environmental benefits. Renewable energy can power electrolysis, a process that splits water molecules into hydrogen and oxygen. This method, known as green hydrogen production, offers a carbon-neutral pathway to produce hydrogen without generating greenhouse gas emissions.
Hydrogen fuel cells have potential applications in various sectors beyond light-duty vehicles, including powering heavy-duty trucks, buses, trains, and even ships. HFCVs offer several advantages over traditional internal combustion engines and battery electric vehicles (BEVs). HFCVs provide longer driving ranges and faster refuelling times, comparable to conventional vehicles, addressing concerns about range anxiety and infrastructure readiness. However, transitioning to a hydrogen-based mobility ecosystem requires substantial infrastructure development. This includes hydrogen production facilities, storage solutions, distribution networks, and refuelling stations. Furthermore, by integrating hydrogen with renewable energy, the mobility sector can significantly reduce its carbon footprint. The lifecycle emissions of hydrogen-based transportation systems, from production to end-use, are substantially lower compared to fossil fuels. This shift not only mitigates air pollution and enhances urban air quality but also contributes to global efforts to achieve carbon neutrality and fulfil climate commitments outlined in international agreements like the Paris Agreement.
Governments and private sectors worldwide are investing in infrastructure to support hydrogen adoption, paving the way for economic growth, job creation, and technological innovation in the energy and transportation sectors. As of 2020, China is the world’s largest producer and consumer of hydrogen, followed by the United States. Global hydrogen production is expected to grow significantly in the coming decades, driven by increasing demand for clean energy solutions. The global hydrogen market, in terms of revenue, was valued at $242.7 billion in 2023 and is projected to reach $410.6 billion by 2030, growing at a CAGR of 7.8% during that period. This growth is driven by favourable regulations, financial incentives, and increased adoption of hydrogen technologies across various industries. Countries like Japan, South Korea, and Germany are considered leaders in hydrogen technology development and deployment for fuel cell vehicles. Africa also has vast renewable energy resources, making it a potential future leader in green hydrogen production. However, major infrastructure development is needed.
As electrolysis technologies mature, low-carbon intensity hydrogen is becoming more competitive compared to conventional hydrogen sources. This cost reduction is a significant driver for the broader adoption of hydrogen across various sectors. Advancements in material science, engineering, and manufacturing have led to increased efficiency and cost-effectiveness of electrolysis-based technologies. Innovations in alkaline, proton exchange membrane (PEM), and solid oxide electrolysers play a major role in achieving these improvements. These developments are pivotal for enhancing the scalability and affordability of clean hydrogen production with low carbon intensity. Furthermore, as policies continue to support technology development in this field, we can expect further progress in hydrogen production methods. Despite its potential, the widespread adoption of hydrogen faces challenges, including high production costs, technological barriers, and the need for coordinated policies and regulatory frameworks. Addressing these challenges requires collaborative efforts from governments, industry stakeholders, and research institutions to drive innovation, lower costs, and scale up hydrogen infrastructure globally.
In conclusion, integrating hydrogen with renewable energy sources presents a transformative opportunity for creating a greener mobility ecosystem. By leveraging hydrogen’s clean energy attributes and renewable energy’s scalability, society can accelerate the transition towards sustainable transportation solutions. This approach not only addresses climate change but also enhances energy security, economic resilience, and environmental stewardship for future generations. Embracing hydrogen as a cornerstone of the mobility revolution underscores our commitment to a cleaner, more sustainable future.
References
Agarwal, R. (2022). Transition to a Hydrogen-Based Economy: Possibilities and Challenges. Sustainability, 14(23), 15975. https://doi.org/10.3390/su142315975
Association (FCHEA), F. C. and H. E. (2021). Hydrogen and Fuel Cell Economy Could Support Millions of Jobs by 2050. Www.prnewswire.com. https://www.prnewswire.com/news-releases/hydrogen-and-fuel-cell-economy-could-support-millions-of-jobs-by-2050-301268110.html
CGEP, C. |. (2021a, June 17). Hydrogen Fact Sheet: Production of Low-Carbon Hydrogen. Center on Global Energy Policy at Columbia University SIPA | CGEP. https://www.energypolicy.columbia.edu/publications/hydrogen-fact-sheet-production-of-low-carbon-hydrogen/
Everything you need to know about hydrogen in the clean energy transition. (n.d.). World Economic Forum. https://www.weforum.org/agenda/2023/01/hydrogen-clean-energy-transition-2023/
Franco, A., & Giovannini, C. (2023). Recent and Future Advances in Water Electrolysis for Green Hydrogen Generation: Critical Analysis and Perspectives. Sustainability, 15(24), 16917–16917. https://doi.org/10.3390/su152416917
Hassan, Q., Sameer Algburi, Aws Zuhair Sameen, Marek Jaszczur, & Hayder Mahmood Salman. (2023). Hydrogen as an energy carrier: properties, storage methods, challenges, and future implications. Environment Systems and Decisions. https://doi.org/10.1007/s10669-023-09932-z
IEA. (2019a, June). The Future of Hydrogen. IEA; IEA. https://www.iea.org/reports/the-future-of-hydrogen
Kim, J. W., Boo, K. J., Cho, J. H., & Moon, I. (2014, January 1). 1 – Key challenges in the development of an infrastructure for hydrogen production, delivery, storage and use (A. Basile & A. Iulianelli, Eds.). ScienceDirect; Woodhead Publishing. https://www.sciencedirect.com/science/article/abs/pii/B9780857097682500018
Nussgruber, C. (2024). Council Post: Top 5 Green Hydrogen Trends For 2024: Paving The Way For A Sustainable Future. Forbes. https://www.forbes.com/sites/forbestechcouncil/2024/04/09/top-5-green-hydrogen-trends-for-2024-paving-the-way-for-a-sustainable-future/
Osman, A. I., Mehta, N., Elgarahy, A. M., Hefny, M., Al-Hinai, A., Al-Muhtaseb, A. H., & Rooney, D. W. (2021). Hydrogen production, storage, utilisation and environmental impacts: a review. Environmental Chemistry Letters, 20(1). https://doi.org/10.1007/s10311-021-01322-8
U.S. Department of energy. (2023). Hydrogen Production: Electrolysis. Energy.gov. https://www.energy.gov/eere/fuelcells/hydrogen-production-electrolysis
US EPA, O. (2015, September 22). Hydrogen in Transportation. Www.epa.gov. https://www.epa.gov/greenvehicles/hydrogen-transportation